Importance of Power Control in Telecommunication Systems
The foundation of modern communication is telecommunications systems, which allow voice, data, and video to be transmitted over long distances. For reliable operation, uninterrupted service, and energy efficiency, these systems predominantly rely on power control. Advanced power control techniques are becoming more and more crucial as the demand for high-speed, high-capacity networks rises and telecommunication infrastructure gets more complex. In addition to ensuring that networks run continuously, efficient power management in telecommunication systems also helps the industry become more sustainable and cost-effective.
Role of Power Control in Telecommunication Systems
Ensuring Reliability and Uptime: It is expected of telecommunication networks to operate with high reliability, frequently needing uptime of close to 100%. In order to achieve this reliability, power control is essential since even minor power outages can cause service disruptions, which can result in large financial losses and dissatisfied customers. Power control systems in telecommunications oversee the distribution and management of electrical power across the network, ensuring that all important components receive a consistent and uninterrupted power supply. This includes backup power options that supply power instantly in the case of a grid failure, including batteries and uninterruptible power supplies (UPS).
Optimizing Energy Efficiency: In telecommunications systems, energy efficiency is a major challenge, especially as network infrastructure expands to handle increasing data traffic and the introduction of new technologies like 5G. In order to optimize energy consumption across telecommunication networks, power control systems are necessary. They accomplish this by adding energy-saving modes during periods of low traffic, lowering power to idle or underutilized equipment, and dynamically modifying power usage based on network demand. These strategies lower operational costs, reduce energy waste, and reduce the damaging effects of telecommunications operations on the environment.
Supporting High-Density Networks: High-density deployments are a hallmark of modern telecommunication networks, especially in metropolitan regions where there is a concentrated demand for data services. In order to manage the energy demands of multiple small cells, base stations, and distributed antenna systems (DAS), high-density networks, which include those used in 5G, require precise power control. Power control systems in such scenarios must balance the need for high performance with the restrictions of space and power availability, ensuring that each network components works efficiently without overloading the power supply or creating excessive heat generation.
Managing Power in Remote and Off-Grid Locations: Numerous telecommunication networks are deployed in isolated or off-grid places, like mountaintops, rural areas, or offshore installations. Power control is essential in these situations to manage limited energy supplies, which are frequently provided by diesel generators or renewable energy sources like solar or wind. These places have advanced power control systems that maximize the use of available power, guarantee the efficient operation of telecommunication equipment, and prolong the life of energy storage systems. They also make remote monitoring and management possible, which enables operators to modify power settings and address issues without requiring on-site intervention.
Challenges Addressed by Power Control in Telecommunications
Heat Management and Cooling: Telecommunications equipment generates heat during operation, which needs to be properly managed to avoid overheating and equipment failure. By controlling power consumption and allocating energy in a way that reduces thermal accumulation, power control systems are essential to heat management. For example, power control can involve integrating intelligent cooling systems that adapt in real time to the network equipment's thermal profile or sequentially switching on power-hungry components to prevent simultaneous peak loads.
Scalability and Flexibility: To handle future growth and technological innovations, telecommunication networks must be adaptable and scalable. By allowing the addition of new network elements without necessitating an extensive overhaul of the existing power infrastructure, power control systems are designed to accommodate this scalability. This is accomplished using modular power systems that can be expanded or reconfigured as needed. Furthermore, power control systems have the ability to adapt to changing network conditions, such as varying traffic loads or the integration of new services, ensuring that the network remains efficient and reliable under various operating conditions.
Minimizing Downtime and Maintenance: Downtime in telecommunication networks can have major financial and reputational ramifications. Power control systems reduce downtime by offering fault-tolerance and redundancy features including load balancing to avoid overloading any one component or automatic switchover to backup power sources. By enabling remote monitoring and diagnostics, these systems help lessen the need for manual maintenance by enabling operators to identify and resolve power-related issues before they cause interruptions in service.
Supporting Next-Generation Technologies: The move to next-generation technologies, such as 5G and beyond, puts additional strain on telecommunication power systems. The enhanced data rates, reduced latency, and extensive device connectivity that these technologies offer necessitate better power densities, more efficient energy management, and increased reliability. Power control systems are crucial in addressing these needs, since they provide the infrastructure needed to power next-generation networks while preserving the energy efficiency and reliability required for sustainable operation.
Future Trends in Power Control for Telecommunications
Integration with Smart Grids: The integration of telecommunication networks with smart grids is becoming more and more popular as these networks continue to develop. The efficiency and reliability of telecommunication power systems can be improved by smart grids' advanced features, which include distributed energy resources (DER) management, demand response, and real-time energy monitoring. Telecommunication companies can minimize their power consumption, take part in grid stabilization initiatives, and more efficiently utilize renewable energy sources by integrating smart grids.
Energy Harvesting and Renewable Integration: The future of power control in telecommunications will probably rely more on renewable energy sources and energy harvesting technologies. Energy from the environment, such as solar, wind, or kinetic energy, can be captured by these systems and converted into usable power for telecommunication equipment. In order to manage the integration of these renewable sources, balance their intermittent nature with the network's continuous power demands, and guarantee that energy storage technologies are used effectively, power control systems will be essential.
Artificial Intelligence and Automation: Artificial intelligence (AI) and automation are projected to play an increasingly essential role in telecommunications power control. AI-powered power management systems are able to evaluate enormous volumes of network data, predict trends in power consumption, and make modifications in real time to enhance reliability and energy efficiency. Additionally, by enabling more responsive and adaptable power regulation, automation can minimize the need for manual intervention and enhance the telecommunication network's overall performance.
Advanced Power Conversion Techniques for Telecommunication Infrastructure
Telecommunication infrastructure is the foundation of modern communication networks, allowing for the transmission of voice, data, and multimedia information over long distances. The power requirements for telecommunication systems have grown dramatically along with the growing demand for faster more reliable communication. The efficient, reliable, and sustainable operation of telecommunication infrastructure relies on advanced power conversion techniques, especially as networks grow and incorporate new technologies like 5G, cloud computing, and edge computing.
High-Efficiency Power Supplies
The power supply system, which converts electrical power from the grid into the specific voltage and current levels required by telecommunication equipment, is one of the most crucial components of the infrastructure. High-efficiency power supplies are required to reduce energy losses and ensure the system's reliability.
Switch-Mode Power Supplies (SMPS): In telecommunications systems, switch-mode power supplies (SMPS) are frequently utilized because of their high efficiency, compact size, and capacity to deliver consistent power output under a variety of load conditions. The SMPS functions by switching power transistors on and off at high frequencies, transforming AC input power into regulated DC output power. The power supply units' size and weight are decreased by using smaller inductors and capacitors due to the high-frequency functioning of SMPS. Furthermore, as compared to traditional linear power supplies, SMPS can attain efficiencies of over 90%, which drastically lowers energy consumption and heat generation.
Digital Power Control: The performance and flexibility of telecommunication infrastructure have been significantly improved by the integration of digital control techniques in power supplies. In order to precisely control voltage regulation, power factor correction, and load balancing, digital power control systems employ microcontrollers or digital signal processors (DSPs) to oversee the operation of power converters. In addition to offering innovative features including remote management and fault detection, this digital approach enables real-time monitoring and adjustment of power supply parameters, increasing efficiency and stability.
Power Factor Correction (PFC)
A crucial strategy in telecommunication infrastructure is power factor correction (PFC), which lowers reactive power consumed from the electrical grid and improves power usage efficiency. PFC is crucial for maximizing the power quality of telecommunication systems because a low power factor can result in higher energy losses, higher utility expenses, and stress on the electrical grid.
Active Power Factor Correction: Active Power Factor Correction is a common technique in telecommunication systems, especially in high-power applications including data centers and base stations. Active PFC circuits use power electronic components such as MOSFETs and IGBTs, which are controlled by dedicated PFC controllers, to shape the input current waveform to match the input voltage. This produces a power factor close to unity, which reduces reactive power and total harmonic distortion (THD) in the power system. Active PFC lowers energy losses while increasing power conversion efficiency by enhancing the power factor.
Benefits in Telecommunication Systems: Several advantages result from the implementation of Active PFC in telecommunication infrastructure, such as lower heat generation, lower energy consumption, and increased power supply system reliability. PFC also aids in meeting regulatory requirements for electromagnetic compatibility (EMC) and power quality, guaranteeing that telecommunication equipment performs within the required limits for noise emissions and harmonic distortion.
Table 1: Benefits of PFC
Reduced Energy Losses | By improving the power factor, active PFC reduces losses in the electrical distribution system, leading to less wasted energy |
Lower Utility Costs | Since utilities often charge based on both real and reactive power, improving the power factor can reduce electricity bills |
Enhanced System Capacity | A higher power factor reduces the need for oversized equipment like transformers, cables, and generators. This increases the capacity of the electrical system |
Compliance with Regulations | Active PFC helps in meeting regulatory standards and power factor requirements, avoiding penalties from utility providers |
Minimized Harmonics | Active PFC reduces harmonic distortion, improving the quality of the power supply and preventing issues with sensitive equipment |
Improved Equipment Lifespan | Reducing harmonics and improving power factor can extend the life of electrical components such as motors and transformers |
Better Voltage Stability | Active PFC helps stabilize voltage levels, reducing the risk of voltage fluctuations that can harm equipment or cause downtime |
Increased System Efficiency | Active power factor correction improves the overall efficiency of the power system by ensuring optimal energy usage |
Lower Heat Generation | A higher power factor reduces the reactive power in the system, leading to less heat generation and better performance in devices like power supplies |
Uninterruptible Power Supplies (UPS)
Uninterruptible Power Supplies (UPS) are an essential component of telecommunication infrastructure because they provide backup power in the case of a grid failure or power outage. UPS systems guard against service interruptions and data loss by ensuring that telecommunication equipment continues to function during power outages.
Online UPS Systems: Online UPS systems, also known as double-conversion UPS, are widely employed in telecommunication facilities because of their ability to deliver continuous and clean power. An online UPS system charges the battery and powers the inverter by first converting the input AC power to DC. The inverter converts the DC back to AC, delivering a consistent and controlled output to the telecommunication equipment. This double-conversion method protects the equipment against power disturbances such as voltage sags, surges, and harmonic distortion, providing consistent operation even in adverse grid conditions.
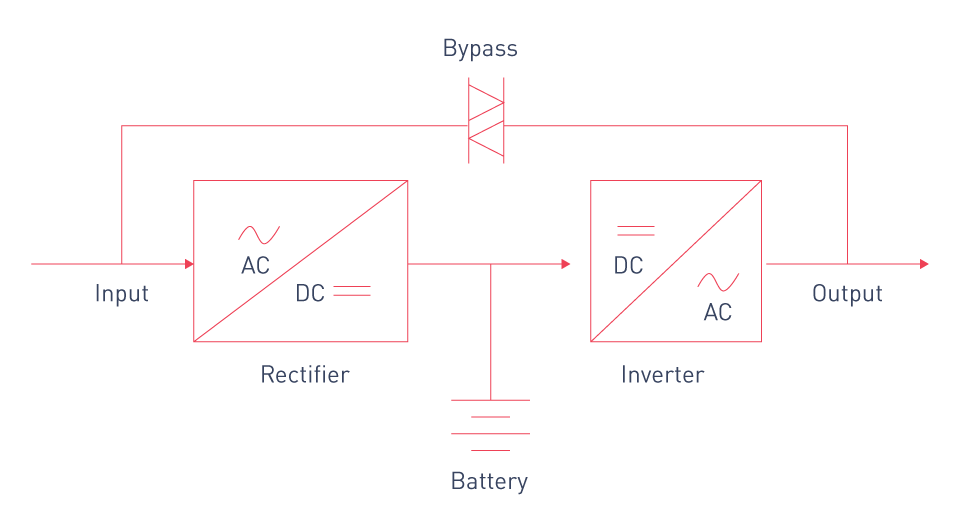
Figure 12: Online UPS
High-Efficiency UPS Designs: High-efficiency UPS systems that reduce energy losses during normal operation and battery backup mode have been developed as a result of advancements in power electronics. Advanced power conversion technologies are incorporated into these UPS systems. Furthermore, sophisticated UPS control systems have the ability to dynamically modify operating parameters in response to load conditions, increasing efficiency and lowering the telecommunication facility's overall consumption of energy.
Energy Storage Systems
Energy storage systems (ESS) are becoming more and more crucial to telecommunication infrastructure as networks grow and the need for continuous uptime increases. By offering energy management, load balancing, and backup power, ESS make sure that telecommunication networks continue to operate even during periods of high demand and grid failures.
Battery Energy Storage Systems (BESS): To provide reliable backup power, telecommunication infrastructure frequently uses Battery Energy Storage Systems (BESS). Because of their high energy density, longer cycle life, fast response times, lithium-ion batteries are the most popular option for BESS. The BESS's power conversion systems control how the batteries are charged and discharged, making sure they are prepared to supply electricity when needed. In order to maximize battery performance and prolong battery life, advanced battery management systems (BMS) monitor the batteries' temperature, state of health (SoH), and state of charge (SoC).
Integration with Renewable Energy Sources: To provide a hybrid power solution, energy storage systems are combined with renewable energy sources including wind turbines or solar panels in telecommunication facilities. Power conversion systems control the energy flow between the grid, energy storage, and renewable sources, guaranteeing consistent and reliable power for telecommunication equipment. In addition to improving the telecommunication infrastructure's sustainability, this integration lowers the facility's overall carbon footprint and acts as a buffer against grid instability.
Cooling and Thermal Management
Effective thermal management is crucial for ensuring the reliability and efficiency of power conversion systems in telecommunication infrastructure. Advanced cooling systems have become increasingly important as equipment gets smaller and power densities rise.
Active Cooling Systems: Fans and liquid cooling are examples of active cooling systems that are commonly used to dissipate heat generated by power conversion components. Because liquid cooling systems can remove heat more efficiently than air cooling, they are particularly effective in high-power applications. In order to maintain optimum operating conditions, prevent thermal shutdowns, and increase the equipment's lifespan, power control systems monitor the temperature of essential components and modify the cooling parameters.
Table 2: Passive vs. active cooling systems
Aspect | Passive Cooling | Active Cooling |
Principle | Relies on natural heat dissipation through conduction, convection, and radiation without the use of mechanical systems | Uses mechanical systems (like fans, compressors, or pumps) to actively cool the environment or component |
Energy Consumption | No external energy is required, as it relies on natural processes. | Requires external power to run fans, compressors, pumps, etc. |
Cost | Lower initial cost and maintenance due to the lack of moving parts | Higher initial cost and ongoing maintenance due to the use of mechanical components |
Efficiency | Suitable for systems with low heat output or environments with adequate natural airflow | More effective for high heat dissipation needs, ensuring precise control over temperature |
Maintenance | Minimal maintenance, since there are no moving parts or active components | Requires regular maintenance (e.g., cleaning filters, checking fans, etc.) |
Temperature Control | Offers limited control over temperature, primarily relying on ambient conditions | Provides precise temperature control with the ability to cool to specific levels |
Applications | Ideal for small-scale systems, low-power devices, or environments with naturally cool temperatures (e.g., passive house designs, electronics). | Used in systems requiring higher cooling power, such as computer servers, air conditioning, or large machinery. |
Passive Cooling Techniques: To control the heat generated by power conversion systems, passive cooling methods including heat sinks and thermal pads are employed in addition to active cooling. The purpose of these passive components is to disperse heat into the surrounding environment and channel it away from crucial places. To increase the efficiency of passive cooling, power conversion systems are frequently designed with integrated thermal management features, such as optimum airflow paths and strategic placement of heat-generating components.
Future Developments in Telecommunication Power Systems
The rapid advancement of technology and the increasing demand for faster, more reliable, and energy-efficient communication networks are driving a major transition in the telecommunication sector. The future of power management in telecommunications systems will be influenced by a number of significant advancements as these systems get increasingly complicated and include new technology. Enhancing energy efficiency, incorporating renewable energy sources, using AI and ML to manage power more intelligently, and adapting to the expanding demands of 5G and beyond are the main goals of these advancements.
Energy Efficiency and Sustainable Power Solutions
The energy consumption of telecommunication infrastructure has grown to be a major concern as telecommunication networks expand. In order to accommodate the increasing demand for data while reducing the environmental impact, future power system developments will place a higher priority on sustainability and energy efficiency.
High-Efficiency Power Conversion: The development of high-efficiency power conversion systems that minimize energy losses and lower the overall power consumption of telecommunication equipment will be one of the key areas of focus. Silicon carbide (SiC) and gallium nitride (GaN) are examples of wide bandgap (WBG) semiconductors that will be essential to increasing power conversion processes' efficiency. These materials enable higher switching rates and lower conduction losses, allowing power supplies, inverters, and other power conversion systems to function more efficiently and reliably.
Green Energy Integration: Future telecommunication power systems will incorporate more renewable energy sources, such as solar and wind power, into their infrastructure. By offering backup power sources in the event of a grid failure, this integration will improve energy resilience while also lowering the carbon footprint of telecommunication networks. Ensuring a steady and uninterrupted power supply to essential telecommunication equipment will require advanced power management systems to regulate the energy flow between the grid, renewable sources, and energy storage systems.
Energy Harvesting: Energy harvesting, which entails obtaining ambient energy from the environment, such as solar, thermal, or radio frequency (RF) energy, and converting it into usable electrical power, is another promising advancement in sustainable power solutions. Energy harvesting could be utilized in telecommunication networks to power low-power equipment such as small cell towers and remote sensors, which would minimize the demand for traditional power sources and improve network sustainability.
AI and Machine Learning in Power Management
A major development in the operation of telecommunication infrastructure is the incorporation of machine learning (ML) and artificial intelligence (AI) into power management systems. AI and machine learning technologies provide better, more adaptive power management systems that can optimize energy usage, forecast and prevent failures, and improve overall telecommunication network efficiency.
Predictive Maintenance and Fault Detection: Large volumes of data collected from telecommunication equipment such as power supplies, inverters, and batteries can be analyzed using AI and ML algorithms. These algorithms can predict potential equipment failures and initiate maintenance procedures prior to a failure by spotting patterns and abnormalities in this data. In addition to lowering maintenance expenses and downtime, this predictive maintenance approach increases the equipment's reliability and lifespan.
Table 3: Benefits of AI and ML for telecom power system management
Benefit | Description |
Predictive Maintenance | AI and ML algorithms analyze historical data to predict equipment failures, enabling proactive maintenance and reducing downtime |
Real-Time Monitoring & Control | AI and ML allow for continuous monitoring of power systems in real-time, providing insights into performance and enabling immediate corrective actions when necessary |
Fault Detection & Diagnostics | ML algorithms can detect faults in power systems earlier by identifying patterns and anomalies, improving the speed and accuracy of diagnostics |
Network Optimization | AI can optimize power distribution networks by balancing load and minimizing losses, enhancing the overall efficiency of telecom infrastructure |
Smart Power Distribution | AI can intelligently control power distribution, balancing demand and ensuring that telecom systems get the right amount of energy at the right time |
Dynamic Power Optimization: AI-powered power management systems have the ability to dynamically modify telecommunication equipment power consumption in response to current network conditions and demand. For instance, AI algorithms can lower base station and other infrastructure power output to save energy during periods of low network traffic. On the other hand, these systems can optimize power distribution during periods of peak demand to guarantee that all essential equipment operates at optimum efficiency. The overall efficiency of telecommunication networks is increased and energy waste is decreased due to this dynamic power usage management.
Smart Grid Integration: AI and machine learning will also play a significant role in the integration of telecommunication networks and smart grids. AI is used by smart grids to better control electricity distribution, balancing supply and demand in real-time. Telecommunication power systems integrated with smart grids can take part in demand response programs, which alter power use based on grid conditions. The influence of power fluctuations on telecommunication infrastructure can be minimized by this integration, which could result in more reliable and efficient power systems.
Adaptation to 5G and Beyond
Significant advancements in power management systems will be required for the deployment of 5G networks and the future development of telecommunication technology beyond 5G. In order to accommodate the enormous number of connected devices and the high-speed data transmission demanded by applications like autonomous vehicles, smart cities, and the Internet of Things (IoT), these new networks require to be more reliable, have lower latency, and consume less power.
5G Power Requirements: 5G networks are predicted to require more power than previous generations of telecommunication networks due to the increased density of small cell sites, the need for high-frequency millimeter-wave transmission, and the widespread usage of Massive MIMO (Multiple Input, Multiple Output) technology. Future power systems need to be extremely efficient, able to manage enormous power loads, and designed with energy efficiency in mind in order to meet these difficulties. In order to avoid overheating 5G equipment that is packed closely together, advanced cooling and thermal management solutions will also be essential.
Edge Computing and Power Distribution: Edge computing, which moves data processing and storage closer to the end-user, will become more important as telecommunication networks advance toward 5G and beyond. A more distributed power infrastructure will be needed for this transition, with power management systems that can accommodate numerous edge data centers and microgrids. To guarantee continuous operation in remote or decentralized locations, these systems will need to be extremely durable and equipped with cutting-edge power conversion and energy storage technologies.
Emerging Technologies for 6G: As we move past 5G, the development of 6G networks will present telecommunication power systems with both new opportunities and challenges. With applications in fields like holographic communications, advanced artificial intelligence, and quantum computing, 6G is expected to enable even faster data rates, exceptionally low latency, and extensive connectivity. In order to meet the massive data processing demands of these cutting-edge applications, 6G power management systems will need to be far more efficient. To fulfill the power demands of 6G networks, innovative power conversion technologies and the use of next-generation materials like graphene will be essential.
Enhanced Reliability and Resilience
The demand for improved power system resilience and reliability will only grow as telecommunication networks become increasingly crucial to the functioning of modern society. Future advancements in power management will concentrate on making sure that the infrastructure supporting telecommunication can endure and recover from various kinds of disturbances, such as equipment failures, cyberattacks, and natural disasters.
Redundant Power Systems: Future power systems will include energy storage solutions and redundant power supplies that can take over in the case of a primary power source failure in order to improve the reliability of telecommunication networks. Advanced control systems that can automatically switch between power sources without affecting service will oversee these redundant systems. Further layers of resilience will be added by microgrids and decentralized power generation, enabling telecommunication networks to function even in the event of widespread grid failures.
Disaster Recovery and Emergency Power: Telecommunication networks have to keep operating in the event of a disaster, such as a hurricane, earthquake, or cyberattack, in order to facilitate emergency communications and recovery efforts. Advanced disaster recovery features including automatic power rerouting, rapid deployment of mobile power units, and priority power allocation to crucial infrastructure will be incorporated into future power management systems. The purpose of these systems is to guarantee that communication networks can quickly recover from interruptions and continue offering essential services.
Cybersecurity in Power Systems: As telecommunication power systems become more connected and integrated with smart grids and IoT devices, cybersecurity will be an increasing concern. Future improvements in power management will involve comprehensive cybersecurity safeguards to protect against cyberattacks that could disrupt power supplies or threaten the integrity of telecommunication networks. Power systems will be shielded from emerging cyber threats by these safeguards, which will include encryption, intrusion detection systems, and secure communication protocols.
Case Study: Energy Management in Data Centers and Networking Equipment
The foundation for modern digital communication is comprised of data centers and networking equipment, which support everything from enterprise operations and telecommunication to cloud computing and internet services. For data center operators and network infrastructure providers, efficient energy management has become a top priority as the need for data processing and storage keeps increasing. This case study explores the methods and tools utilized to maximize energy management in networking equipment and data centers, emphasizing the challenges, solutions, and advantages of implementing advanced power management systems.
Overview of Data Center Energy Consumption
Data Center Growth: Globally, the number and scale of data centers have increased exponentially as a result of the widespread use of cloud computing, big data analytics, and the Internet of Things (IoT). Thousands of computers, storage devices, and networking equipment are housed in these facilities, requiring considerable amounts of power. Since a significant amount of operating expenses is related to the energy required to power and cool these systems, data center operators put a high priority on energy efficiency.
Energy Demand: With power usage usually ranging from a few megawatts for smaller facilities to hundreds of megawatts for hyperscale data centers, data centers are known for their high energy consumption. Servers, storage devices, networking equipment, and the cooling systems necessary to maintain ideal operating temperatures are the primary cause of energy demand. Effective power management techniques are necessary since energy costs are frequently among the biggest operating costs for data centers.
Implementing Energy-Efficient Power Systems
High-Efficiency Power Supplies: Using high-efficiency power supplies for servers and networking equipment is one of the first steps in increasing data centers' energy efficiency. Power supplies with 80 PLUS Platinum (89% efficiency at full load) or Titanium (91% efficiency at full load) efficiency ratings are made to reduce energy losses while converting grid-supplied AC power into the DC power needed for electronic devices. These high-efficiency power supplies minimize heat generation and overall data center energy consumption by lowering conversion losses, which additionally decreases the strain on cooling systems.
Uninterruptible Power Supplies (UPS): When the grid fails or there is a power fluctuation, data centers rely on Uninterruptible Power Supplies (UPS) to provide backup power. In order to reduce losses and guarantee reliable operation, modern UPS systems are designed with energy efficiency in mind, incorporating advanced power conversion technologies. Double-conversion UPS systems, for example, provide a clean and stable power output by constantly converting incoming AC power to DC and back to AC. In order to further reduce consumption of energy, most latest UPS systems also have energy-saving features like "eco mode," which enables the UPS to function in a bypass mode under normal conditions.
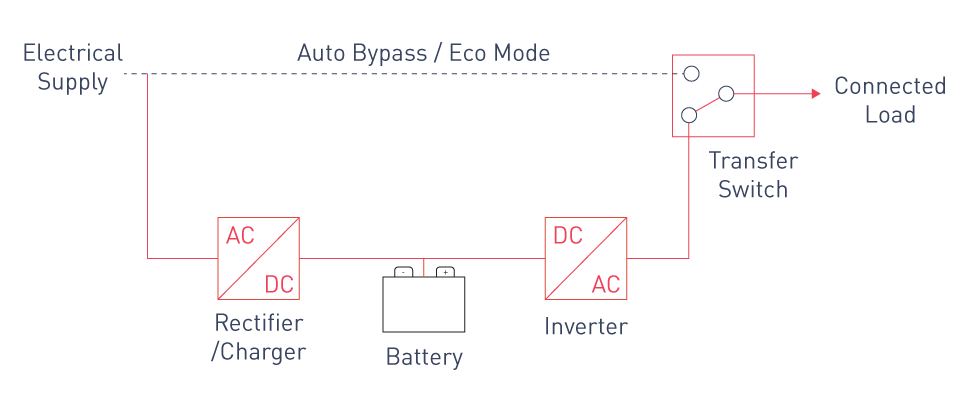
Figure 13: Online UPS with eco mode
Power Distribution Units (PDUs): Efficient power distribution is crucial for lowering energy losses in data centers. PDUs, or intelligent power distribution units, monitor and control how electricity is distributed to servers, storage devices, and networking equipment. By giving operators access to real-time power usage data, these PDUs enable them to identify inefficiencies and improve power distribution. Intelligent PDUs can remotely regulate individual outlets, allowing operators to decrease power usage during off-peak hours or switch off unused equipment.
Table 4: Normal vs. intelligent PDUs
Feature | Normal PDU | Intelligent PDU |
Function | Distributes power to multiple devices in a rack | Distributes power with added monitoring and control features |
Power Monitoring | No power monitoring capabilities | Provides real-time monitoring of power usage (voltage, current, load, etc.) |
Control Features | No remote control or switching of outlets | Remote control for turning outlets on/off, and outlet-level power cycling |
Network Connectivity | Typically not connected to a network | Can connect to a network (via Ethernet, Wi-Fi) for remote management |
Remote Management | No remote access or management | Remote management via web interface, SNMP, or other protocols |
Energy Efficiency | No power-saving features | Can optimize energy usage and provide insights into energy efficiency |
Usage in Data Centers | Suitable for basic applications where monitoring is not critical | Ideal for data centers, server rooms, or environments requiring high uptime and energy efficiency |
Cooling and Thermal Management
Cooling Challenges: Cooling is one of the most energy-intensive processes in data centers, accounting for up to 40% of overall energy consumption. Managing heat generation gets more difficult as processor power and server densities rise. Hotspots, equipment failures, and higher operating expenses can result from inefficient cooling systems.
Innovative Cooling Solutions: Data centers are implementing innovative cooling solutions that lower energy consumption while preserving ideal operating temperatures in order to address these challenges. For instance, liquid cooling eliminates the requirement for traditional air conditioning systems by using chilled liquid to absorb heat directly from servers and networking equipment. Because it removes heat more effectively than air cooling, this approach is very efficient, especially for high-density server setups.
Table 5: Convection vs. liquid cooling
Feature | Convection Cooling | Liquid Cooling |
Cooling Method | Relies on air to dissipate heat through natural or forced convection | Uses liquid to transfer heat away from equipment |
Efficiency | Less efficient at cooling high-density racks due to air's lower thermal conductivity | More efficient, as liquid has a higher heat capacity and conductivity than air |
Energy Consumption | Requires fans to circulate air, consuming energy but less than liquid cooling systems | Requires pumps and cooling systems, consuming more energy than air-based systems |
Installation Complexity | Relatively simple to install with minimal infrastructure | More complex to install, requiring piping, pumps, and a cooling infrastructure |
Space Requirements | Requires larger space for airflow management and AC units | Requires additional space for piping and cooling units, but can be more compact in dense setups |
Maintenance | Lower maintenance needs but periodic cleaning of filters or fans may be required. | Requires more frequent maintenance for pumps, coolant refills, and checking for leaks |
Application | Best suited for low to mid-density server environments | Ideal for high-density server environments or situations where maximum cooling efficiency is required |
Airflow Management: Another crucial aspect of data center cooling is efficient airflow management. Data centers can minimize the stress on cooling systems and improve overall energy efficiency by optimizing airflow across server racks and minimizing hot air recirculation. In order to keep the facility's temperature constant and avoid overheating, strategies like hot aisle/cold aisle containment, in which hot air is released from the back and cold air is directed toward the front of servers, are used.
Renewable Energy Integration and Energy Storage
Renewable Energy Sources: Many data centers are incorporating renewable energy sources including solar and wind power into their electrical infrastructure as part of a larger commitment to sustainability. Data centers can reduce their carbon footprint and dependency on fossil fuels by producing clean energy on-site or by acquiring renewable energy credits. Incorporating renewable energy also strengthens the power supply's stability and acts as a hedge against growing electricity costs.
Energy Storage Systems (ESS): Data centers are rapidly implementing Energy Storage Systems (ESS) to optimize the usage of renewable energy sources and provide a steady power supply. These systems, which are often based on lithium-ion batteries, store extra energy produced by renewable sources while demand is low and release it when demand is high. In the event of a grid failure, ESS also supplies backup power, guaranteeing the continuous operation of critical data center. In order to maximize efficiency, advanced energy management systems balance the use of stored energy, renewable energy, and grid power while optimizing the charging and discharging of ESS.
Case Study: Energy Management in a Hyperscale Data Center
A hyperscale data center run by a major technological company is an excellent example of advanced energy management in action. With hundreds of thousands of servers and billions of transactions daily, this facility is among the biggest in the world. The data center has implemented a thorough energy management strategy that incorporates innovative cooling solutions, high-efficiency power supplies, and integration with renewable energy sources in order to handle the massive energy demand.
Power Supply Optimization: Power supplies with 80 PLUS Titanium certification are utilized in the data center, guaranteeing an extremely efficient energy conversion process. These power supplies increase the facility's overall energy efficiency by minimizing energy losses and lowering the generation of heat. Furthermore, modular UPS systems with eco-mode capabilities are used in the data center, allowing substantial energy savings during normal operation.
Cooling and Thermal Management: To manage the heat generated by its tightly packed servers, the data center has implemented liquid cooling technology. This approach lowers the facility's overall consumption of energy and eliminates the need for traditional air conditioning. Additionally, the data center uses advanced airflow management techniques, including hot aisle/cold aisle containment, to guarantee that cooling is distributed efficiently and evenly across the entire facility.
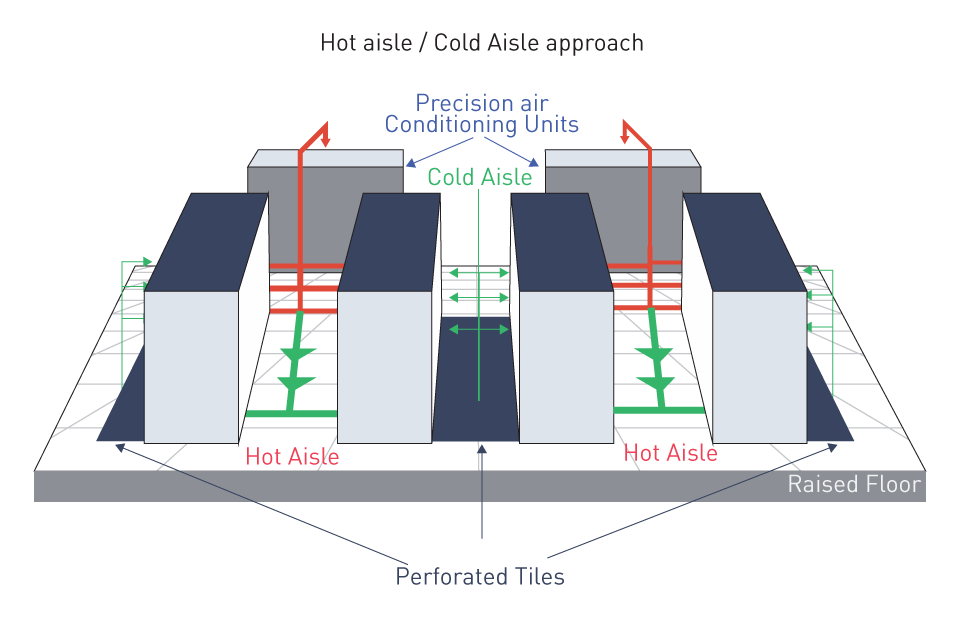
Figure 14: Hot aisle/cold aisle containment
Renewable Energy and Storage: A significant portion of the data center's energy needs are met by renewable sources via a combination of off-site wind farms and on-site solar panels. A large-scale Energy Storage System (ESS) facilitates the integration of renewable energy by storing excess energy generated during periods of low demand and supplying it during periods of peak usage. In addition to improving the data center's sustainability, this strategy offers a reliable backup power source in the event of grid failures.
Results: The energy management technique employed at the hyperscale data center has resulted in a significant decrease in energy usage and operational costs. One of the most energy-efficient data centers in the world, the facility operates with a Power Usage Effectiveness (PUE) ratio significantly lower than the industry average because to the utilization of high-efficiency power supplies, innovative cooling solutions, and renewable energy integration.
$$ \text{PUE} = \frac{\text{Total Facility Energy Usage}}{\text{IT Equipment Energy Usage}} $$The performance of this energy management approach highlights how modern power management systems can drive sustainability and efficiency in the fast-developing data center industry.
直接登录
创建新帐号