Overview of Power Electronics in Consumer Devices
Power electronics are essential to the operation and performance of modern consumer devices. Power electronics are in charge of effectively converting and controlling electrical energy to satisfy the unique needs of each device, from laptops and smartphones to home appliances and entertainment systems. The significance of advanced power electronics in consumer devices is becoming increasingly apparent as consumer demand for gadgets that are smaller, more powerful, and use less energy keeps rising.
Role of Power Electronics in Consumer Devices
Energy Conversion: Most consumer devices rely on the conversion of electrical energy from one form to another. Battery-operated gadgets, for instance, need power electronics to transform the battery's DC voltage into the different voltage levels required by the device's components. Power electronics are also necessary for devices that plug into AC outlets in order to transform the AC voltage into appropriate DC levels. Power supplies, adapters, or integrated power management circuits usually handle this energy conversion process to ensure the device operates efficiently and reliably.
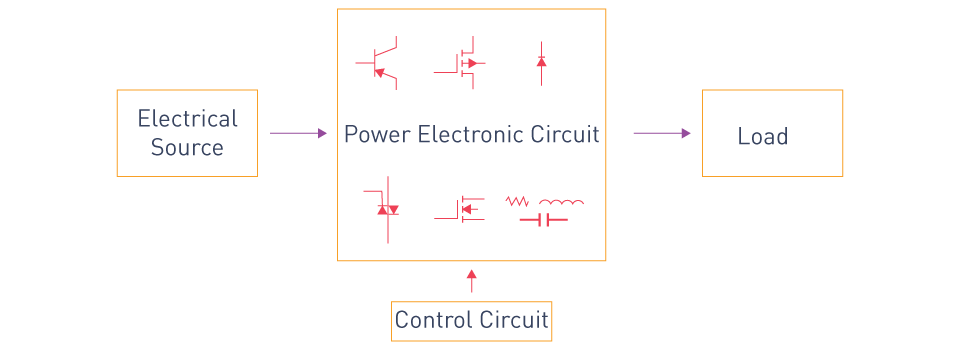
Figure 1: Energy conversion
Voltage Regulation: Voltage regulation is one of the primary functions of power electronics in consumer electronics. Because electronic components are often sensitive to fluctuations in voltage levels, voltage management is essential for ensuring stable performance. Power electronics circuits, including switch-mode power supplies (SMPS) or linear regulators, make sure that even when the input power fluctuates, the voltage delivered to every component of the device stays within the required range. This regulation improves the device's overall performance and lifespan while preventing component damage.
Power Management: Power electronics in consumer electronics are in charge of controlling the device's total power usage in addition to energy conversion and voltage regulation. The energy consumption of the device's components is optimized according to their current operational requirements using advanced power management techniques such as power gating, which cuts off power to circuit blocks that are not in use, and dynamic voltage scaling, which modifies a component's voltage in real-time based on workload. For example, in order to preserve battery life, a smartphone may lower the amount of power delivered to its processor while it is in standby mode. These power management techniques are essential for lowering the energy consumption of home electronics and prolonging the battery life of portable devices.
Common Power Electronics Components in Consumer Devices
Power Adapters and Chargers: Power adapters and chargers are among the most common applications of power electronics in consumer devices. These devices transform wall outlet AC electricity into the DC power needed by portable devices including tablets, laptops, and smartphones. Switch-mode power supplies (SMPS) are commonly used in modern converters and chargers due to their high efficiency, compact size, and ability to operate across a wide variety of input voltages. Additionally, these chargers have power control capabilities that guard against battery overcharging and overheating.
Battery Management Systems (BMS): The Battery Management System (BMS) is essential to the safe and efficient operation of battery-powered devices, including wearable gadgets, laptops, and smartphones. The BMS regulates the charging and discharging procedures, monitors the battery's State of Charge (SoC) and State of Health (SoH), and protects against issues like thermal runaway, undervoltage, and overvoltage. The BMS enhances the device's overall reliability and user experience by maximizing the battery's performance and lifespan.
DC/DC Converters: It is necessary to utilize DC/DC converters because many consumer gadgets require different voltage levels for different components. The voltage from the battery or power supply is step up or step down to the required levels by these converters. In a laptop, for instance, a DC/DC converter may step up the voltage to power the display backlight while stepping down the battery voltage to power the processor. To maximize energy efficiency and guarantee that every component receives the appropriate power level, DC/DC converters are crucial.
Inverters and Converters in Home Appliances: Power electronics regulate the operation of motors and compressors in home appliances including air conditioners, washing machines, and refrigerators. In order to improve performance and energy efficiency, inverters and converters are frequently used to modify motor speed in accordance with load requirements. For example, an inverter air conditioner reduces energy consumption and wear on its components since the compressor speed is continually regulated to maintain the correct temperature rather than cycling on and off.
Trends in Power Electronics for Consumer Devices
Miniaturization: Miniaturized power electronics components are growing more and more in demand as consumer gadgets get smaller and more compact. Smaller, more efficient power electronics that can manage higher power densities are becoming possible because to developments in semiconductor technology, such as the development of wide bandgap materials like silicon carbide (SiC) and gallium nitride (GaN). Manufacturers can now fit more functionality into smaller devices without compromising on performance or energy efficiency because of the miniaturization trend.
Energy Efficiency: Energy efficiency is a top priority in the design of power electronics for consumer gadgets. Manufacturers are investing in the development of power electronics that lower energy usage during active use and standby modes in response to growing consumer and regulatory demand for energy-efficient products. Devices are incorporating strategies including intelligent power management, low-power standby modes, and high-efficiency power conversion to meet energy efficiency requirements and prolong battery life.
Wireless Charging and Energy Harvesting: Wireless charging technology is becoming more common in consumer electronics, removing the need for physical connectors and allowing for more convenient charging options. For wireless charging devices to transmit and convert electricity efficiently, power electronics are necessary. Furthermore, methods for energy harvesting, which collect and transform ambient energy from sources including light, heat, and motion, are being explored as a means of supplying low-energy devices, like wearables and sensors, without the need of batteries.
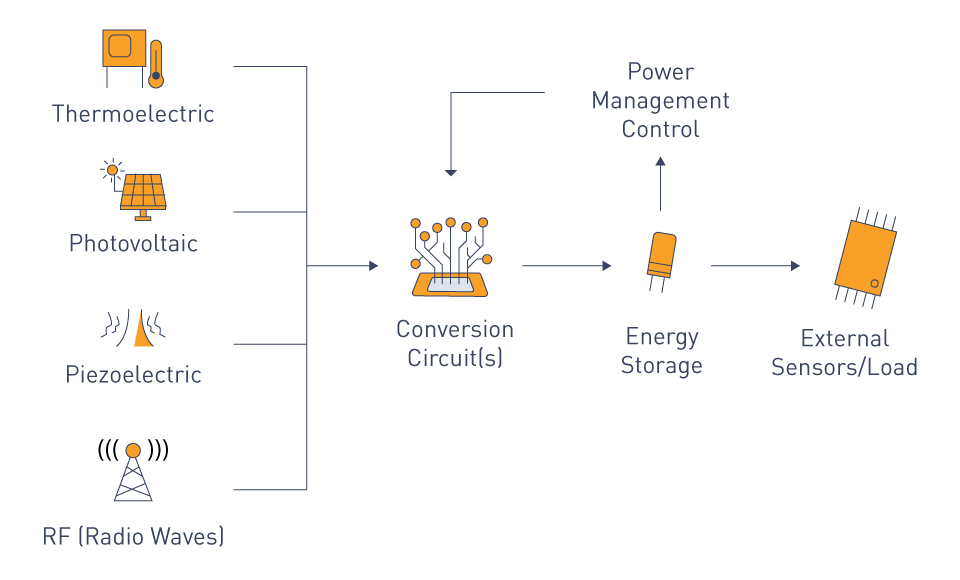
Figure 2: Energy harvesting
Smart Devices and IoT: Power electronics now faces both new opportunities and challenges as a result of the widespread use of smart devices and the Internet of Things (IoT). For these devices to operate well in a networked environment, they need to be continuously connected and have minimal power consumption. Power electronics are being developed to meet the specific power needs of Internet of Things devices, such as low-power modes, energy harvesting capabilities, and energy-efficient communication protocols. The need for advanced power electronics in consumer electronics is expected to increase as the Internet of Things expands.
Role of Control Systems in Enhancing Device Performance and Efficiency
Control systems are essential to maximizing the efficiency and performance of consumer electronics, including wearables, laptops, tablets, and smartphones. The demand for advanced control strategies in power electronics is growing as consumers demand greater functionality, longer battery life, and improved user experiences. These control systems are intended to handle different aspects of device operation, including power consumption, thermal management, and dynamic resource allocation, all of which are required to achieve the necessary performance while being energy efficient.
Power Management
Controlling power consumption is one of the main purposes of control systems in consumer electronics. This is accomplished by employing techniques including adaptive power management, power gating, and Dynamic Voltage and Frequency Scaling (DVFS), which modify the power consumption of various components in response to demands in real time.
Dynamic Voltage and Frequency Scaling (DVFS): DVFS is a common technique in which the voltage and frequency provided to a processor are changed based on the workload. For instance, the control system raises the voltage and frequency to guarantee optimal performance while a smartphone is engaged in demanding activities like gaming or streaming videos. On the other hand, the control system lowers the voltage and frequency to conserve energy and prolong battery life during inactivity or less demanding tasks. Through dynamic power consumption optimization, DVFS helps in striking a balance between energy efficiency and performance.
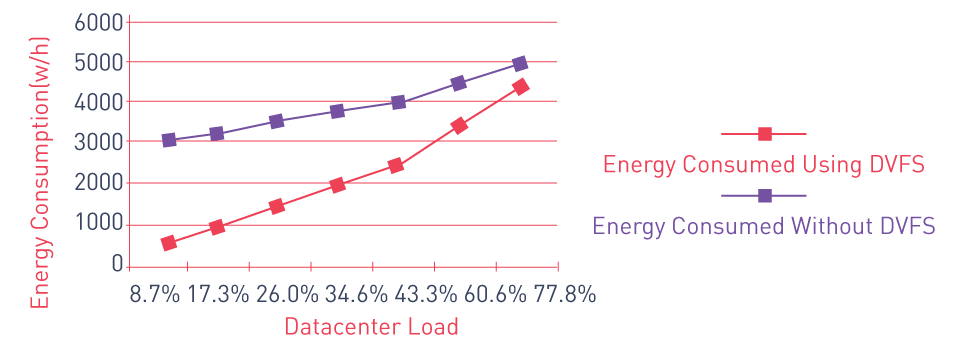
Figure 3: Datacenter energy savings with DVFS
Power Gating: Power gating is the process of turning off circuit components while not in use. The total power consumption of gadgets like laptops can be decreased by turning off specific parts, like the GPU or specific processor cores, while not in use. Control systems ensure that the process is smooth and does not affect the user experience by managing the changes between active and inactive states.
Adaptive Power Management: Real-time data and algorithms are used by adaptive power management systems to accurately predict and control power usage. By adjusting power consumption across multiple components to optimize battery life without compromising performance, these systems can adjust to the user's behavior and environmental conditions. For instance, adaptive power management in wearable devices can modify sensors and display power consumption in response to user activity and ambient light conditions.
Thermal Management
Controlling the heat generated by high-performance components becomes more crucial as consumer devices get compact and more powerful. Thermal management, which keeps equipment operating within safe temperature limits while preserving performance, relies heavily on control systems.
Active Cooling Control: Active cooling systems with fans or liquid cooling are used to dissipate heat in devices such as high-performance laptops. Control systems monitor the temperature of crucial components, including the CPU and GPU, and modify the cooling systems as necessary. These systems aid in maintaining the ideal balance between noise levels and cooling efficiency by dynamically regulating fan speeds or turning on liquid cooling only when required.
Thermal Throttling: A technique known as "thermal throttling" reduces a device's performance when the temperature rises above a certain threshold. In order to prevent damage and minimize heat generation, control mechanisms that detect overheating temporarily restrict the processor's speed. Although it could result in decreased performance during intensive tasks, this serves to ensure user safety and prolong the device's lifespan.
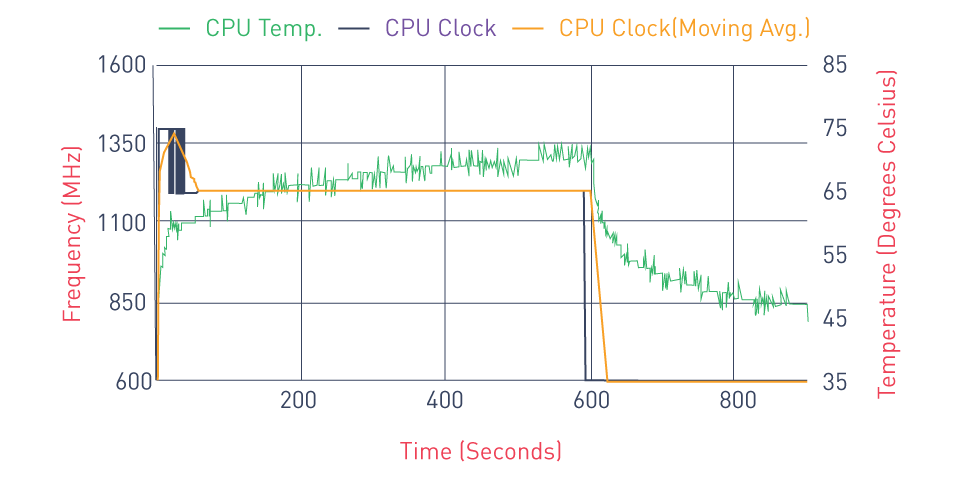
Figure 4: Raspberry Pi thermal throttling
Passive Cooling and Heat Dissipation: Passive cooling techniques like heat sinks and thermal pads are frequently utilized in smaller devices, such as wearables and smartphones. In order to reduce heat generation, control systems in these devices optimize the distribution of tasks across components. Workload distribution algorithms, for example, can distribute the computational load and prevent any one component from overheating, hence lowering the need for intense thermal management techniques.
Battery Management
In consumer electronics, battery management systems (BMS) are essential, particularly for portable devices that use rechargeable batteries. BMS control systems are responsible for monitoring battery health, controlling cycles of charging and discharging, and optimizing power consumption to prolong battery life.
State of Charge (SoC) and State of Health (SoH) Monitoring: To provide precise information on the battery's remaining life and overall health, control systems constantly monitor the battery's SoC and SoH. Through this monitoring, the device can make informed decisions about power distribution and notify users when charging is needed or when battery health is deteriorating.
Battery Charging Control: To extend battery life, advanced control systems regulate the charging process. Fast charging is one example of this, in which the control system modifies the charging current to reduce charging time while avoiding overcharging and overheating. To lessen battery wear, smart charging algorithms also modify the charging procedure in response to variables like battery temperature and usage patterns.
Power Allocation: Control systems regulate the distribution of power in devices with numerous power-hungry components, including smartphones with multiple cameras, displays, and processors. For example, in order to maximize battery usage during video recording, the control system may prioritize power delivery to the camera and CPU while lowering power to less crucial components.
Dynamic Resource Allocation
Modern consumer electronics frequently have to perform multiple tasks at once, necessitating dynamic resource allocation for optimal performance and seamless operation. Control systems are essential for controlling how resources, including CPU time, memory, and power, are allocated to various tasks.
Load Balancing: To maximize efficiency and performance, control systems divide tasks among multiple cores or processors. These systems keep the computational load balanced such that no single core overheats or becomes a bottleneck, ensuring steady performance on all tasks.
Task Prioritization: Control systems prioritize tasks in multitasking environments according to their urgency and significance. For instance, with a smartphone, foreground tasks like playing videos may be given more resources while background tasks like app updates can be deprioritized to preserve power. Even when several tasks are underway, this prioritizing makes sure that the user experience is responsive and seamless.
Real-Time Optimization: To adjust to changing conditions, control systems also use real-time optimization techniques. For instance, the control system reallocates resources to prioritize graphics processing and lowers power to less important functions when a user turns from reading an article to playing a game. This dynamic adjustment maximizes power consumption while preserving a high level of performance.
Challenges and Future Directions in Consumer Electronics Power Management
As consumer electronics become more powerful, compact, and integral in daily life, power management is becoming a crucial area of focus. From laptops and smartphones to wearables and smart home appliances, effective power management is necessary to optimize performance, prolong battery life, and guarantee user satisfaction. However, as these gadgets become more sophisticated, the power management challenges increase. In order to overcome these challenges, innovative approaches and an optimistic perspective on the development of power electronics in consumer electronics are needed.
Challenges in n Consumer Electronics Power Management
Increased Power Density: The power density of consumer electronics increases as they get smaller and more feature rich. More power is needed in a limited area due to the demand for lighter, more compact gadgets with more processing power, higher-resolution displays, and advanced connectivity features (including 5G). Because overheating can reduce component lifespans and impair device performance, managing this increased power density presents significant thermal management challenges. Power management systems must be designed by engineers to effectively distribute and dissipate heat while preserving device reliability.
Battery Life and Energy Efficiency: One major issue with portable consumer electronics is still battery life. Even while gadgets are becoming more power-hungry due to larger screens, high-performance processors, and always-on connectivity, users still expect an extended battery life. It is difficult to strike this balance between energy efficiency and performance. In order to optimize energy consumption, power management systems must dynamically modify power supply according to the operational status of the device. For example, they may use low-power modes while the device is not in use or reduce power to idle components.
Complex Power Management Systems: Complex power management systems that can manage multiple power domains, each with varying voltage and current requirements, are frequently needed for modern consumer devices. For instance, the processor, display, memory, and radio frequency (RF) circuits of a smartphone may each have their own power domain. The design of power management becomes more complex when various power domains are coordinated to maximize overall energy efficiency while guaranteeing that every component gets the power it needs. Managing unpredictable power inputs becomes even more difficult when renewable energy sources, such as solar-powered wearables, are integrated.
Thermal Management: Efficient thermal management is critical for device performance and reliability. Traditional cooling methods including fans and heat sinks have less space as devices get thinner and more compact. Because of this, dissipating the heat produced by high-performance components is challenging. To efficiently manage heat without making devices heavier or larger, engineers must explore advanced cooling techniques such as phase-change cooling, advanced materials, or passive cooling approaches.
Regulatory and Environmental Considerations: Consumer electronics are subject to severe energy efficiency regulatory requirements, such as the Energy Star program and the European Union's Ecodesign Directive. In some areas, devices must adhere to these requirements in order to be sold, which might make power management design challenging. The need for sustainable power management solutions that minimize energy usage and decrease electrical waste is also driven by the increased consumer demand for environmentally friendly products. Engineers have to strike a compromise between the requirement for power management innovation and regulatory compliance.
Future Directions in Consumer Electronics Power Management
Advanced Battery Technologies: Future consumer devices will probably have advanced battery technologies that provide more energy density, quicker charging times, and longer lifespans in order to address the ongoing challenge of battery life. For instance, solid-state batteries offer increased energy storage capacity and improved safety, which are major advantages over traditional lithium-ion batteries. Furthermore, new chemistries and materials like graphene-based or lithium-sulfur batteries have the potential to completely transform energy storage in consumer devices.
Wireless Power Transfer and Energy Harvesting: Many consumer devices currently have wireless charging built-inin. Nonetheless, advancements in wireless power transfer may make this function more widely used and efficient in the future. Devices may be able to charge wirelessly over greater distances or even while in use thanks to methods like radio frequency (RF) energy harvesting and resonant inductive coupling. Energy harvesting technologies, which gather ambient energy from sources like light, heat, or motion, could also be integrated into consumer gadgets, lowering dependence on traditional charging methods and improving battery life.
Artificial Intelligence (AI) in Power Management: AI and machine learning are projected to become increasingly significant in power management for consumer electronics. AI-drive power management systems are able to optimize energy use in real time by analyzing usage patterns and environmental conditions. AI could, for example, predict when a user is most likely to use the device and modify the power settings accordingly to save energy. Through better power distribution management and charging cycle optimization, machine learning algorithms can potentially contribute to increased battery performance.
Ultra-Low Power Electronics: The development of ultra-low power electronics is crucial for the next generation of consumer gadgets, notably in IoT and wearable technologies. Innovations in low-power circuit design, energy-efficient microprocessors, and advanced power management techniques are crucial since these devices frequently need to operate on minimal power for extended periods of time. Research into sub-threshold circuits, energy-efficient computing architectures, and power-efficient communication protocols will propel the development of devices that can harvest energy from their surroundings or operate for years on a single battery.
Integration of Renewable Energy Sources: Future consumer devices may include flexible-wearable solar cells and other renewable energy sources more frequently into their designs. This is particularly important for wearables, outdoor devices, and Internet of Things sensors that might not have easy access to traditional power sources. Sophisticated power management systems that can efficiently convert and store energy from renewable sources while guaranteeing a consistent power supply to the device's components are necessary for integrating renewable energy.
Flexible and Wearable Electronics: Stretchable and flexible power management solutions are becoming more and more necessary as consumer demand for wearable devices grows. Wearable technology that is both comfortable and energy-efficient will be made possible by advancements in flexible batteries, stretchable electronics, and energy storage materials. These developments will be essential for powering wearable devices that need constant power in a small, flexible form factor, such as smart clothes and next-generation health monitoring devices.
Case Study: Consumer Electronics Utilizing Advanced Control Techniques
Consumer electronics, especially laptops and smartphones, have completely changed how they manage power, improve performance, and deliver user experiences through the integration of advanced control techniques. This case study examines how advanced control systems, including dynamic power management, thermal regulation, and intelligent resource allocation, have been incorporated into modern laptops and smartphones. By examining such examples, we can gain a better understanding of how important control systems are to solving the complex challenges that today's high-performance consumer electronics face.
Dynamic Power Management in Smartphones
One of the best examples of how advanced control techniques can maximize power usage without sacrificing performance can be observed in smartphones. These gadgets need to maintain a long battery life while juggling the demands of various sensors, wireless connectivity, high-resolution displays, and powerful processors.
Dynamic Voltage and Frequency Scaling (DVFS): The dynamic voltage and frequency scaling (DVFS) technique is one of the key techniques used in smartphones. Depending on the workload, this method enables the processor to modify its operating voltage and frequency. For example, the control system reduces the voltage and frequency to conserve power when performing a basic operation like web browsing. In contrast, the voltage and frequency are increased to guarantee smooth operation during resource-intensive tasks like video editing or gaming. Battery life is significantly extended by this dynamic modification while preserving the required performance levels.
Application-Specific Power Management: Application-specific power management techniques are used by modern smartphones, in which the control system optimizes power consumption according to the particular requirements of the application that is active. For example, the control system lowers power to other less important components when the user is watching a movie, giving the GPU and display priority. By doing this, the battery is used effectively, and the video playback is smooth.
Battery Management Systems (BMS): Smartphones use sophisticated battery management systems that monitor the battery's state of charge (SoC) and health (SoH). By using this data, the BMS can prolong the battery's life, avoid overcharging, and optimize charging cycles. To ensure safe and efficient charging, adaptive charging algorithms, for example, modify the charging speed in response to the battery's temperature and usage patterns.
Thermal Regulation in Laptops
Laptops, specifically high-performance models used for professional applications or gaming, produce a lot of heat when operating. Preventing overheating and preserving performance require effective thermal management. Laptops' advanced control systems use both active and passive cooling techniques to regulate temperature.
Active Cooling with Intelligent Fan Control: Fans are usually the primary active cooling method in laptops. Using real-time temperature data from sensors placed on the CPU, GPU, and other crucial components, advanced control systems dynamically modify fan speeds. For instance, the fan speed is lowered in low-power modes to save noise and power consumption, while the fan speed is increased during a gaming session, to dissipate heat more effectively. Some high-end laptops also use liquid cooling systems, in which the control system regulates the coolant flow to essential components according to their thermal and temperature load.
Thermal Throttling: Laptops use thermal throttling, in which the control system lowers the clock speed of the CPU or GPU when temperatures get near the critical levels, to avoid overheating. Even though the laptop's performance is reduced, this technique guarantees that it will continue to function safely. Thermal throttling is especially crucial for prolonged high-performance tasks where heat accumulation can be substantial, such gaming or video processing.
Passive Cooling and Heat Dissipation: To disperse heat away from crucial components, laptops also employ passive cooling techniques like heat pipes and thermal pads. Aggressive thermal management is not necessary because the control system distributes workloads optimally to avoid any one component overheating.
Intelligent Resource Allocation in Multi-Tasking Environments
The ability to multitask is becoming more and more necessary for both laptops and smartphones, from running applications in the background to processing in real time for productivity, gaming, and video calling. To guarantee smooth operation, advanced control systems are crucial for controlling the distribution of resources including CPU time, memory, and power.
Load Balancing and Task Prioritization: Control systems in multi-core processors dynamically distribute tasks among different cores in order to balance the load and prevent any one core from becoming a bottleneck. The control system, for instance, distributes resources to guarantee that the video editing process has enough processing power without interfering with other operations while a laptop is running a video editing program, streaming music, and downloading files. Algorithms for task prioritization ensure that important tasks are completed first, improving the user experience overall.
Real-Time Performance Optimization: To optimize resource allocation, advanced control systems continuously assess the device's performance and make adjustments in real time. To provide seamless operation, the control system, for example, reallocates resources from low-priority background processes to the augmented reality (AR) application when a smartphone transitions from standby mode to running the AR application. In dynamic contexts, this real-time optimization is essential for sustaining high performance.
Case Study: Apple’s A-Series Processors in iPhones
One of the best examples of how advanced control techniques are incorporated into consumer devices is Apple's A-series processors, which are utilized in iPhones. These CPUs have specifically designed efficiency and performance cores that collaborate to dynamically control both performance and power consumption.
Performance and Efficiency Cores: High-performance and high-efficiency cores are both present in the A-series processors. Based on their demands, the control system automatically distributes tasks to the proper cores. The efficiency cores are utilized for low-demand activities like texting or checking email to save battery life. The performance cores are turned on to supply the required processing power for more demanding tasks like video editing or gaming.
Neural Engine: The Neural Engine, a specialist component designed for machine learning tasks, can also be integrated with the control system. The Neural Engine dynamically distributes resources according to real-time requirements, optimizing AI-related tasks like image processing and facial recognition. Because of this, the iPhone can efficiently complete complicated tasks without exhausting all of its battery.
Battery and Thermal Management: The A-series processors have advanced battery and thermal management systems. To avoid overheating and extend battery life, the control system modifies the charging rate and task distribution based on battery health and charging patterns. Thermal management algorithms regulate the distribution of tasks among processor cores to reduce heat generation while maintaining peak performance.
直接登录
创建新帐号